Basics of oxidation and reduction
村上定瞭(水浄化フォーラム), Sadaaki Murakami (Water & Solutions Forum)
1.物質間での電子移動
2.反応の自由エネルギー変化
3.酸化還元電位とは
(1) pHと電位
(2) 標準酸化還元電位
(3) 酸化還元電位のまとめ
4.酸化還元電位の具体例
(1)金属の酸化還元電位
(2)各物質の酸化還元電位
5.酸化還元反応の速度
6.水質測定の事例
7.水浄化の事例
8.電極の機能と名称
1. Electron transfer between substances
2. Free energy change of the reaction
3. What is redox potential?
(1) pH and potential
(2) Standard redox potential
(3) Summary of redox potential
4. Specific examples of redox potential
(1) Redox potential of metal
(2) Redox potential of each substance
5. Rate of oxidation-reduction reaction
6. Example of water quality measurement
7. Example of water purification
8. Function and name of electrode
1.物質間での電子e-の移動
単体・化合物・イオンなどの物質は相互に、その物質中の原子を構成する電子を授受して安定な状態を保っている。電子の移動反応では、放出する物質Red(電子供与体または還元剤)と受け取る物質Ox(電子受容体または酸化剤)の両物質が必ず存在する(Red:reductant、Ox:oxidant、Redox反応-酸化還元反応)。
見かけ上、物質間の電子移動反応は観測することはできないが、酸化還元反応を関与する反応過程を分解することで、その電子移動反応を理解することができる。
例えば、酸性水溶液中で、Fe2+が溶存酸素O2によって、Fe3+に酸化される反応は、次式(1)で示される。
上記の反応過程を分解すると、次式で示される。
O2 + 4H+ + 4e– = 2H2O (1b)
式(1a)に示すように、Fe2+は電子e–を放出して、Fe3+に変化している。一方、O2は4e–を受け取り、4H+と結合して2分子のH2Oに変化していることが理解できる。
なお、酸性における式(1)の反応速度は極めて遅く、pH上昇とともに反応速度は指数関数的に増加するが、Fe2+やFe3+は水酸化物を形成して反応系が複雑となるので、説明を簡単にするために、酸性での反応系を扱うこととする。
1. Transfer of electron between substances
Substances, such as simple molecules, ions and complex compounds, maintain a stable state by exchanging electrons that form the atoms in the substance with each other. In the electron transfer reaction, there is always a substance that emits Red (electron donor or reducing agent) and a substance that receives Ox (electron acceptor or oxidizing agent): Red-reductant, Ox-oxidant; Redox reaction-Red and Ox reaction.
Apparently, the electron transfer reaction between substances cannot be observed, but the electron transfer reaction can be understood by decomposing the reaction process involving the redox reaction.
For example, a reaction in which Fe2+ is oxidized to Fe3+ by dissolved oxygen O2 in an acidic aqueous solution is represented by the following formula (1).
The above reaction process is decomposed into the following equation.
O2 + 4H+ + 4e– = 2H2O (1b)
As shown in the formula (1a), Fe2+ emits an electron e− and changes to Fe3+. Meanwhile, O2 receives 4e– and combines with 4H+, resulting in that two molecules of H2O is produced.
Incidentally, the reaction rate of the formula (1) in acidic is very slow, the reaction rate will increase exponentially with increase in pH, but the reaction systems of Fe2+ and Fe3+ are extremely complicated because of formations of hydroxide compounds in neutral and alkaline water. Therefore, in order to simplify the explanation, we will deal with acidic reaction systems.
2.反応の自由エネルギー変化 ΔG
物質Xの自由エネルギーGXは、次式で示される。
(μX:化学ポテンシャル、aX:活量)
反応(1)の自由エネルギー変化ΔGは、
= 4GFe3+ + 2GH2O – 4GFe2+ – GO2 – 4GH+ (3a)
= ΔG’°+ RTln K’
= ΔG°+ RTln K (3b)
(式(3a)に、式(2)を代入)
ΔG’° = 4μ°Fe3+ + 2μ°H2O – 4μ°Fe2+ – μ°O2– 4μ°H+
K’ = (aFe3+)4(aH2O)2/((aFe2+)4(aO2)(aH+)4)
(水の活量aH2Oは一定とする)
ΔG° = ΔG’° – 2RTln aH2O
K = (aFe3+)4/((aFe2+)4(PO2)(aH+)4) (3c)
pK = 4paFe3+ – 4paFe2+ – pPO2 – 4paH+
pK = 4pFe3+ – 4pFe2+ – pPO2 – 4pH (3d)
(K:平衡定数、pX = –log[X])
(希薄溶液:aX = fX[X] = [X]、fX = 1)
(PX:気体の分圧(atm))
今、化学反応はΔGが減少する方向へ進行するので、反応式(1)において、自由エネルギー変化ΔGの式(3~3d)の関係より、
(a) Gleft > Gright すなわち 4pFe2+ + pPO2 + 4pH < pFe3+ のとき、右方向へ反応が進行する(例えば、Fe2+濃度・酸素分圧PO2の増加やpHの低下)。
(b) Gleft < Gright すなわち 4pFe2+ + pPO2 + 4pH > pFe3+ のとき、左方向へ反応が進行する(例えば、無酸素状態やpHの上昇)。
(b)において、他の酸化剤を投入するか、白金などの不活性な一対の電極を浸して電圧を加えるなどでは、Gleftに外部エネルギーGoutが加わり、Gleft + Gout > Grightとなれば、右方向へ反応が進行することとなる。
なお、水浄化における具体的な電極反応プロセスおよび浄化プロセスの設計・制御に重要な電位-pHの平衡図については、別のページで解説する。
2. Change in free energy of reaction ΔG
Free energy GX of substance X is shown by the following equation.
(μX : chemical potential, aX: activity)
The free energy change ΔG of reaction(1) is
= 4GFe3+ + 2GH2O – 4GFe2+ – GO2 – 4GH+ (3a)
= ΔG’°+ RTln K’
= ΔG°+ RTln K (3b)
(Substituting equation (2) into equation (3a))
ΔG’° = 4μ°Fe3+ + 2μ°H2O – 4μ°Fe2+ – μ°O2– 4μ°H+
K’ = (aFe3+)4(aH2O)2/((aFe2+)4(aO2)(aH+)4)
(Water activity aH2O is constant.)
ΔG° = ΔG’° – 2RTln aH2O
K = (aFe3+)4/((aFe2+)4(PO2)(aH+)4) (3c)
pK = 4paFe3+ – 4paFe2+ – pPO2 – 4paH+
pK = 4pFe3+ – 4pFe2+ – pPO2 – 4pH (3d)
(K: equilibrium constant, pX = –log[X])
(Dilute solution: aX = fX[X] = [X]、fX = 1)
(PX: partial pressure of gas (atm))
Now, since the chemical reaction progresses in the direction of decreasing ΔG, the reaction(1) will proceed as follows from the relationship of equations(3 to 3d) for the free energy change ΔG.
(a) When Gleft > Gright, that is, 4pFe2+ + pPO2 + 4pH < pFe3+, the reaction proceeds to the right (e.g., Fe 2+ concentration and/or the oxygen partial pressure PO2 increase, and/or the pH reduces).
(b) When Gleft < Gright, taht is, 4pFe2+ + pPO2 + 4pH > pFe3+, the reaction proceeds to the left (for example, anoxic state or increase of pH).
The reaction will proceed to the right in the cace of (b) when another oxidant is added or a pair of inert electrodes such as platinum is dipped to apply a voltage so that the external energy Gout is added to Glight to be Gleft + Gout > Gright.
The potential-pH equilibrium diagram, which is important for the design and control of the specific electrode reaction process for water purification, is explained on another page.
3.酸化還元電位とは
上記の反応過程(1)は、電子を含むイオン反応式(1a)と(1b)に分解すると、物質間の電子移動が理解できることが示された。この分解された反応は、半反応とも呼ばれる。
水溶液中において、物質A、電子e–および水素イオンH+)が関与する半反応は次式で一般化できる。
反応(4)では、電気量zFを伴うから自由エネルギーの変化は、
式(5)は、上記(2)・(3a~d)に示した各関係式と同様に扱い、E°= -ΔG°/(zF)とすると、次式で示される。(正確には、ΔG → ΔG° – c(μ°H2O + RTln aH2O) である。)
K = (aB)b/((aA)a (aH)m))
温度25℃(T=298K)において、定数R(=8.314 J/K/mol)、F(=96,500 C/mol)、ln = 2.303logを用い、式(6)を書き直す。
= E°- 0.0591(m/z)pH – 0.0591(1/z)(apA– bpB) (7)
(Eの単位:V(volt) = J/C)
3. What is redox potential?
It is shown that the above reaction(1) process can be understood by electron transfer between substances when decomposed into ion reactions (1a) and (1b) including electrons. This decomposed reaction is also called a half reaction .
In an aqueous solution, the half reaction involving substance A, electron e– and hydrogen ion H+ can be generalized by the following equation.
In reaction (4), the change of free energy is
Expression (5) is treated in the same manner as the relational expressions shown in (2) and (3a to 3d) above, and if E° = −ΔG°/(zF), the following expression is given.
(To be exact, ΔG → ΔG° – c(μ°H2O + RTln) aH2O).)
K = (aB)b/((aA)a (aH)m))
Rewrite equation (6) using constants R (= 8.314 J/K/mol), F (= 96,500 C/mol), and ln = 2.303 log at a temperature of 25°C (T = 298K ).
= E°- 0.0591(m/z)pH – 0.0591(1/z)(apA– bpB) (7)
(Unit of E:V(volt) = J/C)
(1)pHと電位
(a)m=0
このケースでは、酸化還元電位Eは、 r = apA – bpB = p(Aa/Bb) によって変化する。pHは関与しない。
(b)m≠0
このケースでは、酸化還元電位Eは、前記 r が一定であれば、pHが±1ほど‘増減’すれば、0.059(m/z)[V]ほど‘減増’することとなる。
(1) pH and potential
(a) m = 0
In this case, the redox potential E changes with r = apA – bpB = p(Aa/Bb). pH is not involved.
(b) m ≠ 0
In this case, the oxidation-reduction potential E is “increased” by 0.059 (m/z) [V] when the pH is “decreased” by ±1, if the r above is constant.
(2)標準酸化還元電位 E°
一般に数表に表示されている酸化還元電位は、反応式(4)において、関与する全ての物質Xの活量aX = 1 としたときの値でpX = 0となり、式(7)はE = E°となる。
温度25℃、関与する物質の活量を’1’としたときの電位を標準酸化還元電位という。
なお、酸化還元電位の絶対値を求めることは不可能で、基準となる半反応の電位を‘0’と定め、これに対して目的とする半反応の電位との電位差を測定し、その値を目的とする半反応の酸化還元電位としている。一般的には、標準水素電極SHE(standard hydrogen electrode)の電位を基準としている。
(pH = 1.0、PH2 = 1.0atm)
(2) Standard redox potential E°
Generally, the redox potential shown in the table is pX = 0 when the activity aX = 1 of all involved substances X in the reaction(4), and the formula (7) is E = E°. The potential when the temperature of 25°C and the activity of the substances involved is set to ‘1’ is called the standard redox potential. In addition, it is impossible to obtain the absolute value of the redox potential, and the potential of the reference half-reaction is set to ‘0’, and the potential difference from the potential of the desired half-reaction is measured, and the difference value is determined and defined as the redox potential of the desired half reaction. Generally, the potential of a standard hydrogen electrode SHE is used as a reference.
(pH = 1.0、PH2 = 1.0atm)
(3)酸化還元電位のまとめ
(a) 酸化還元電位の基準
① 式(8)で示す標準水素電極の電位SHEを’0’とする。
② 半反応(4)の電位Eは、SHEに対する電位差[V]で示す。
(b) 物質Xの活量
① 酸化還元反応に関わる各物質Xは活量aXで示す。
② 固体X(s)の活量および水H2Oの活量は’1’とする。
aX(s) = 1 → log aX(s)= 0; aH2O = 1 → log aH2O = 0
③ 物質Xの活量aXは、水溶液中の濃度[X]を用いて表すと、aX = fX[X]の関係があり、fXを活量係数(0<fX≦1)といい、無限希釈([X] → 0)のとき、fX → 1となる。したがって、希薄溶液では、aX = [X]として扱ってもよいこととなる。
(c) 標準酸化還元電位E°
半反応(4)の標準酸化還元電位E°は、温度25℃、反応に関わる全物質の活量aXi = 1のときの、式(6)で示す酸化還元電位Eである。
留意事項
一般的に、標準酸化還元電位E°は、式(5)で示す自由エネルギー変化ΔGから求められている。実際に、電気化学的手法を用いてSHEに対して式(4)の電位Eを測定したとき、式(6)から計算した電位Eと一致しない。電気化学測定においては電解質を加えるので、この電解質との相互作用により反応に関与する物質Xの活量が異なるからである。
電解質を加えない純水中で物質Xを無限に希釈して、電位Eを測定すれば、aX = [X]となり、ΔGから求めた式(5)と実験的に式(6)から求めた電位Eは一致することとなろうが、このような実験系での電位の測定は不可能である。
(3) Summary of redox potential
(a) Redox potential reference
① The potential SHE of the standard hydrogen electrode shown in reaction (8) is set to ‘0’.
② The potential E of the half reaction (4) is indicated by the potential difference [V] with respect to SHE.
(b) Activity of substance X
① Each substance X involved in the redox reaction is shown by activity aX.
② The activity of solid X(s) and the activity of water H2O shall be ‘1’.
aX(s) = 1 → log aX(s) = 0; aH2O = 1 → log aH2O = 0
③ When the activity aX of the substance X is expressed using the concentration [X] in the aqueous solution, there is a relationship of aX = fX[X], and fX is called an activity coefficient (0<fX≦1), which is infinite dilution (when [X] → 0, fX → 1). Therefore, in dilute solution, it can be treated as aX = [X].
(c) the standard redox potential E°
The standard redox potential E° of the half reaction (4) is the redox potential E shown in equation (6), when the temperature is 25°C and the activity of all substances involved in the reaction is aXi = 1.
Notes
In general, the standard redox potential E° is obtained from the free energy change ΔG shown in equation(5). Actually, when the potential E of the reaction(4) is measured with respect to SHE using the electrochemical method, it does not match the potential E calculated from the equation(6). Since an electrolyte is added in the electrochemical measurement, the activity of the substance X involved in the reaction is different due to the interaction with the electrolyte.
If the substance X is diluted infinitely in pure water without adding any electrolyte and the potential E is measured, aX = [X], the E-value obtained theoretically from the equation(5) using ΔG and the E-value experimentally from the equation(6) would be the same, but it is impossible to measure the potentials in such an experimental system abve.
4.酸化還元電位の具体例
(1)金属
(a)金属の酸化還元電位と一般的性質
代表的な金属-金属イオン(水和)の標準酸化還元電位(標準電極電位)を表1に示す。酸化還元電位(以下、Redox電位)が低い金属は外殻電子エネルギーが高く、電子を放出してイオン化する傾向(還元力)が強い。
① Redox電位の低い金属(電子を放出する傾向が極めて高い)
Li~Naは、常温でも空気中の酸素と速やかに反応して酸化物を生成する。水とは常温で反応してH2ガスを発生して、自らはイオンとなり水に溶ける。
② Redox電位が中程度の金属(電子を放出する傾向が中程度)
Mg~Pbは常温では空気中の酸素と反応しにくいが(Mgは比較的反応しやすい。)、放置すると表面に酸化皮膜をつくる。高温では空気中の酸素とよく反応し、MgやAlの粉末を空気中で熱すると、強い光を出して燃え、酸化物 MgOやAl2O3となる。
Mgは熱水と反応し、水酸化物Mg(OH)2を生成する。ZnやFeは高温の水蒸気と反応してH2ガスを発生し、自らは酸化物ZnOやFe3O4となる。
Ni、Sn、Pbは希酸に溶けてH2を発生するが、空気中の酸素との反応は起こりにくい。Niより、Redox電位の高い金属は水とはほとんど反応しない。Pbは不溶性のPbCl2やPbSO4を生成するので、HClやH2SO4にはほとんど溶けない。
③ Redox電位の高い金属(電子を放出する傾向が低い)
水素H2よりもRedox電位が高いCu~Auは空気中の酸素と反応しにくく、希酸とも反応しない。CuやHgは空気中で加熱すると酸化されて酸化物となる。Cu~Agは酸化力のある酸(希硝酸・濃硝酸/熱濃硫酸)とは反応し、NOx/SO2ガスを発生し、自らはイオン化して溶ける。
PtやAuは王水(濃塩酸1+濃硝酸3)のみにイオン化して溶ける。PtやAuは空気中でも安定して存在する。
4. Specific example of redox potential
(1) Metal
(a) Redox potential and general properties of metals
Table 1 shows standard redox potentials (standard electrode potentials) of typical hydrated metal ions. A metal having a low redox potential has a high outer shell electron energy, and has a strong tendency to emit electrons to be ionized (reducing power).
① Metals with low redox potential (very high tendency to emit electrons)
Metal of Li to Na react rapidly with oxygen in the air even at room temperature to form oxides. These metals react with water at room temperature to generate H2 gas to become ions and dissolve in water.
② Metals with a medium redox potential (moderate tendency to emit electrons)
Metals of Mg to Pb do not easily react with oxygen in the air at room temperature (Mg reacts relatively easily), but if left in the air, oxide films are formed on these surfaces. At high temperatures, these react well with oxygen in the air, and when Mg and Al powders are heated in the air, they emit intense light and burn to form oxides MgO and Al2O3.
Mg reacts with hot water to form hydroxide Mg(OH)2. Zn and Fe react with high temperature steam to generate H2 gas, and become ZnO and Fe3O4 oxides.
Ni, Sn, and Pb dissolve in dilute acid to generate H2, but the reaction with oxygen in the air is unlikely to occur. Metals with a higher Redox potential than Ni hardly react with water. Pb is practically insoluble in HCl and H2SO4 because of the formations of PbCl2 and PbSO4 which are insoluble in water.
③ Metals with high Redox potential (low tendency to emit electrons)
Metals of Cu to Au, which have higher redox potentials than hydrogen H2, do not easily react with oxygen in the air and do not react with dilute acid. Cu and Hg are oxidized to oxides when heated in the air. Metals of Cu to Ag react with oxidizing acids (dilute/concentrated nitric acid and hot concentrated sulfuric acid) to respectively generate NOx and SO2 gases, to ionize and dissolve in acids.
Pt and Au are ionized and dissolved only in aqua regia (concentrated hydrochloric acid(1) + concentrated nitric acid(3)). Pt and Au are stable even in the air.
Table 1 Redox potentials of metals and their reactions with oxygen and water.
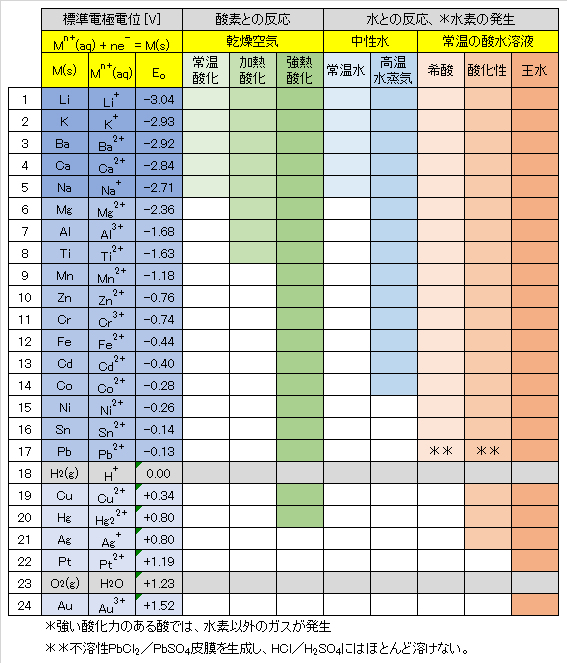
(b)金属と他金属イオンとの反応
図1(A)に示すように、硫酸銅(Ⅱ)CuSO4水溶液に亜鉛板Znを浸漬すると、ZnがZn2+として溶解し、Zn板状上にCuが析出して被膜を形成する。また、図1(B)に示すように多孔質の隔壁(素焼き板)で隔てた両室に硫酸銅(Ⅱ)および硫酸亜鉛(Ⅱ)の各水溶液(希硫酸で酸性とする)を満たし、それぞれにCu板およびZn板を浸漬して、両板をリード線で接続すると電流が流れ、Cu板上にはCuが析出し、Zn板からZn2+が溶出する(ダニエル電池)。
これらの電子移動反応は、つぎのように説明される。なお、簡単のために、各イオンの活量係数を’1’とする。
Cu2+ + 2e– → Cu E = +0.34 + RT/(2F)ln[Cu2+] (10)
外殻電子のエネルギーは、e–(in Zn) > e–(in Cu)であるので、電子はZn→Cuへ移動して安定化する(図2に示すように、電子は高いエネルギー状態から低いエネルギー状態へ移動する。)。すなわち、式(9)で左方向へ、式(10)で右方向へ反応が進行し、理論的にはlog[Zn2+]/[Cu2+]=37(式(9)と式(10)のEが等しくなるとき)に達したときに反応が停止する。
この反応で放出されるエネルギーは図1(A)では溶液の温度上昇、図1(B)では外部抵抗体の発熱エネルギーに変換される。厳密には、一部のエネルギーは溶液中でのイオン移動にも消費され、わずかではあるが溶液の温度上昇を伴う。
電池の表記
図1(B)は、Zn|Zn2+‖Cu2+|Cu 、と簡略表記される。式(9)と(10)に示すように、イオン濃度により電位が変化するので、条件によっては電子の移動方向(電流は逆方向)は異なるが、ECu > EZn の場合には、(-)Zn|Zn2+‖Cu2+|Cu(+)、と表記することもある。’|’は電子移動反応が発生する界面、’‖’は隔膜や塩橋(電解質イオンは透過するが、溶液は混合しない)を示す。Pt(不活性で電子の授受のみに関与)を用いた水素電極SHEとCu電極を塩橋で接続した電池は、Cu|Cu2+‖H+|Pt,H2(1atm)、のように表記される。
留意事項
このページで述べていることは平衡反応(時間単位が含まれていない)であって、電子移動量の速度、すなわち、電流の大きさ(単位時間あたりに流れる電気量または物質の変化量に)については触れていない。これについては、浸漬金属の表面積、反応イオンの濃度とその移動速度、水-金属界面での活性化エネルギーなど、様々な因子が関与するので、別ページで記載する。
(b) Reaction of metal with other metal ions
As shown in Fig.1(A), when a zinc plate Zn is immersed in an aqueous solution of Cu(II) sulfate CuSO4, Zn dissolves as Zn2+ and Cu deposits on the Zn plate to form a film. Further, as shown in Fig.1(B), both chambers separated by a porous partition wall (unbaked plate) are filled with respective aqueous solutions of copper(II) sulfate and zinc(II) sulfate (which are acidified with dilute sulfuric acid), When a Cu plate and a Zn plate are dipped in each, and both plates are connected by a lead wire, an electric current flows, Cu is deposited on the Cu plate, and Zn2+ is eluted from the Zn plate (Daniel battery).
These electron transfer reactions are explained as follows. For simplicity, the activity coefficient of each ion is set to ‘1’.
Cu2+ + 2e– → Cu E = +0.34 + RT/(2F)ln[Cu2+] (10)
Since the energy of the outer shell electron is e–(in Zn) > e–(in Cu), the electron moves from Zn to Cu and stabilizes (As shown in Fig.2, eletron moves to a lower energy state.). That is, the reaction proceeds to the left in Eq.(9) and to the right in Eq.(10), and the reaction stops theoretically when log[Zn2+]/[Cu2+]=37 (E-values in Eqs.(9) and (10) are equal).
The energy released by this reaction is converted into the temperature rise of the solution in Fig.1(A), and is converted into the heat generation energy of the external resistor in Fig.1(B). Strictly speaking in Fig.1(B), some energy is also consumed in moving ions in the solution with a slight increase in the temperature of the solution.
Battery notation
Figure 1(B) is abbreviated as Zn|Zn2+‖Cu2+|Cu. As shown in Eqs. (9) and (10), the potential changes depending on the ion concentration, so the electron transfer direction (current is opposite direction) differs depending on the conditions, but in the case of ECu > EZn, it may also be expressed as (-)Zn|Zn2+|Cu2+|Cu(+). “|” indicates an interface where an electron transfer reaction occurs, and “‖” indicates a diaphragm or a salt bridge (electrolyte ions permeate but a solution does not mix). A battery in which the hydrogen electrode SHE, using Pt (inactive and involved only in the transfer of electrons), and the Cu electrode are connected by a salt bridge is described as Cu|Cu2+‖H+|Pt,H2(1atm).
Notes
What are described on this page are equilibrium reactions (time unit is not included), and the velocities of electron transfers are not mentioned. The magnitude of electric current (in the amount of electricity flowing per unit time or the amount of change in substance) depends on various factors such as the surface area of the electrode metal, the concentrations of reactive ions and their migration rates, and the activation energies at the water-metal interface are involved, so they are described on another page.
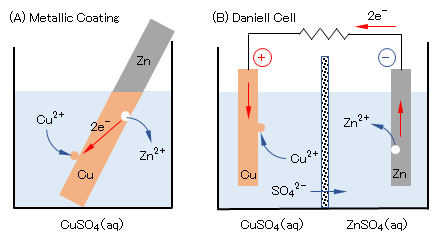
図1 酸化還元電位の異なる金属での電子移動反応
Fig.1 Electron transfer reactions on metals with different redox potentials.
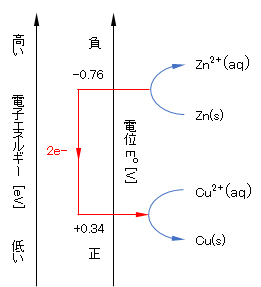
図2 酸化還元系における電子移動反応
Fig.2 Electron transfer reaction in redox system.
(2)様々な物質の酸化還元電位
様々な物質の標準酸化還元電位を表2~7に示す。ここでは、水浄化に関わる酸化還元反応の代表的な事例について、簡単に説明する。なお、下記の電池/電解系の表記法には正式でないものも含まれおり、簡略・平易化のために使用したので注意されたい。
(2) Redox potential of various substances
Standard redox potentials of various substances are shown in Tables 2 to 7. Here, Typical examples of redox reactions related to water purification will be briefly described. Note that some of the battery/electrolysis system notations listed below are not official, but are used for simplicity and simplification.
Table 2 Standard redox potentials of sparingly soluble salts.
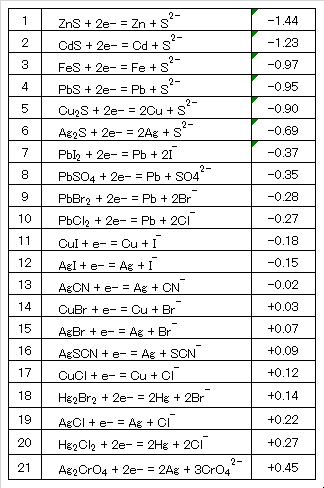
Table 3 Standard redox potentials of metal ions.
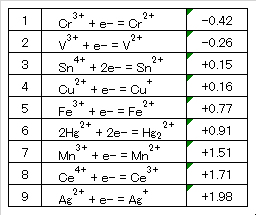
Table 4 Standard redox potentials of metal complex ions.
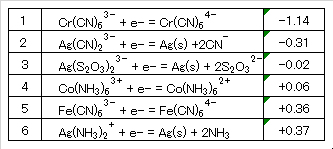
Table 5 Standard redox potentials of sulfur and halogen.
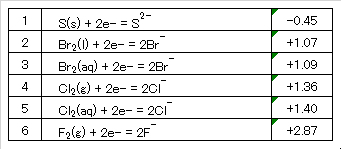
Table 6 Standard redox potentials of inorganic substances.
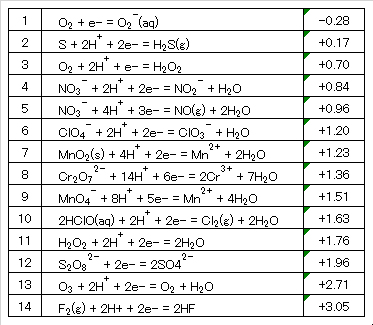
Table 7 Standard redox potentials of organic compounds.
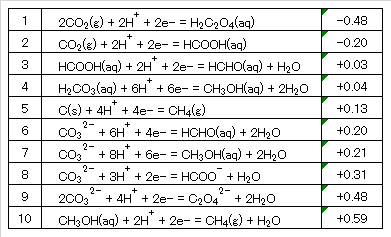
6.水質測定の事例
(a) pHの測定
ガラス膜センサーの両面(検水側 pHx と標準液側 pHs = 7)に発生する電位差ΔEを参照電極を用いて測定し、検水のpHxを測定する。電位差は、高入力インピーダンス電圧計に、つぎに示すAg(+)極およびAg(-)極をリード線で接続して測定する。
検水(t=25℃)のpHx = 7のときΔE = 0となるように電圧計を調整すると、pHx = ・・、6、7、8、・・のとき、ΔE[mV] = ・・、59.1、0.00、-59.1、・・となる。
参照電極には、銀-塩化銀電極(Ag|AgCl/Cl–)(表2-19)が電位の再現性・精度に優れ、取扱いやすいため、多用されている。ガラス膜の電位発生機構については、省略する。
6. Examples of water quality measurements
(a) pH measurement
Measure the pHx of the test water by measuring the potential difference ΔE generated on both sides (pHx on the test water side and pHs = 7 on the standard solution side) of the glass membrane sensor using the reference electrode. The potential difference is measured by connecting the following Ag(+) and Ag(-) electrodes with lead wires to a high input impedance voltmeter.
If the voltmeter is adjusted so that ΔE = 0 at pHx = 7 of the test water (t=25°C), ΔE[mV] = …, 59.1, 0.00, -59.1,…, when pHx = …, 6, 7, 8, …
As the reference electrode, a silver-silver chloride electrode (Ag|AgCl/Cl–) (Table 2-19) is widely used because it has excellent potential reproducibility and accuracy and is easy to handle. The potential generation mechanism of the glass membrane is omitted.
Ag(+)|AgCl/Cl– (reference electrode) ‖Test water (pHx)|Glass membrane|Standard solution (pHs)‖Cl–/AgCl|Ag(-) (reference electrode)
ΔE = -RT/(F)(pHx – pHs) (9)
(b) DO(溶存酸素)の測定
隔膜電極法によるDO測定には、ポーラログラフ式(電気分解、電解)とガルバニ電池式がある。ポーラログラフ式では電極に対し加電圧するための外部電源が必要であるが、ガルバニ電池式では測定系そのものが電池を形成するので加電圧は不要である。
いずれも、電解液(KCl)に浸漬した測定電極(作用電極)(Pt、AuまたはAgなどの不活性な金属)および参照電極(対極)(Ag|AgCl/Cl–、Ag|Ag2O/OH–、Pb|PbO/OH–など)から構成される電解/電池系で、この電解/電池系を酸素を選択的に透過させる隔膜で仕切ったものである。
Pt(+)極とAg(-)極をリード線で電流計に接続する。検水中の酸素は隔膜を透過して上記電解系に入ると、つぎの電解反応がおこり、Ag極からPt極へ電子が移動し、酸素濃度に比例した電流(電子と逆方向)が流れるので、これをDO値に変換する。DOと電圧(電流を電圧に変換)の関係は、無酸素と飽和DOの水溶液で校正しておく。
(b) DO (dissolved oxygen) measurement
DO measurement by diaphragm electrode method includes polarographic method (electrolysis) and galvanic cell method. The polarographic method requires an external power source to apply a voltage to the electrodes, whereas the galvanic battery method does not require an applied voltage because the measurement system itself forms a battery.
In both cases, the measurement electrode (working electrode) (inert metal such as Pt, Au, or Ag) and reference electrode (counter electrode) (Ag|AgCl/Cl–, Ag|Ag2O/OH–) immersed in electrolyte (KCl), Pb|PbO/OH–, etc.), which is partitioned by a membrane that selectively permeates oxygen.
Connect the Pt(+) and Ag(-) electrodes to the ammeter with lead wires. When oxygen in the test water permeates the diaphragm and enters the above-mentioned electrolytic system, the next electrolytic reaction occurs, electrons move from the Ag electrode to the Pt electrode, and a current proportional to the oxygen concentration (the opposite direction of the electrons) flows and convert this value to a DO value. The relationship between DO and voltage (which is converted from current) is calibrated with an oxygen-free and saturated DO aqueous solution.
Ag(-): 4Ag + 4Cl– → 4AgCl + 4e- (e-の放出)
電気化学(電気分解、電池)や電子回路では、外部から/へ、電流i/電子e-が流入/流出する電極をアノード(Anode)、外部へ/から、電流i/電子e-を流出/流入する電極をカソード(Cathode)と呼ぶ。一方、電位で電極の極性を定義するときには、電位が高い方を正極(+)、低い方を負極(-)と呼ぶ。電流は高い方から低い方向へ流れ、電子は低い方から高いほうへ移動する。
電池系(放電)と電気分解系(加電または充電)では電子(または電流)の流れる方向が逆となるので、注意する。
①電気分解: 正極=アノード、負極=カソード、②電池: 正極=カソード、負極=アノード。
「電極の機能と名称」については、本ページ・ボトムに詳細を記載している。
In electrochemistry (electrolysis, battery) and electronic circuits, the electrode where the current i/electron e– inflows/outflows from/to the outside is called anode, and the electrode where the current i/electron e– outflows/inflowsis called a cathode. On the other hand, when defining the polarity of the electrode by the potential, the one with the higher potential is called the positive electrode (+) and the one with the lower potential is called the negative electrode (-). The current flows from high to low, and the electrons move from low to high.
Note that the directions of electrons (or current) flow in the battery system (discharging) and the electrolysis system (charging) are opposite.
① Electrolysis: positive electrode = anode, negative electrode = cathode, ② battery: positive electrode = cathode, negative electrode = anode.
“Function and name of electrode” is described in detail at the bottom of this page.
(c) COD(化学的酸素要求量)の測定
CODは、検水に酸化剤として、過マンガン酸カリウムK2MnO4(表6-9)または二クロム酸カリウムK2Cr2O7(表6-8)を加え、一定の条件下(酸の添加、所定時間の加熱)で有機物と酸化剤を反応させ、反応終了後、残存する酸化剤を定量して、消費された酸化剤の量を求め、この消費量を酸素の量[mg-O2/L]に換算したものである。
残存する酸化剤の定量には、還元剤、例えばシュウ酸カリウムK2C2O4(表7-9)が用いられる。
(c) Measurement of COD (chemical oxygen demand)
For COD, potassium permanganate K2MnO4 (Table 6-9) or potassium dichromate K2Cr2O7 (Table 6-8) is added to the test water as an oxidizing agent under certain conditions (addition of acid, heating for a predetermined time). After reacting the organic substance with the oxidant added and quantifying the remaining oxidant after the reaction, the amount of consumed oxidant is calculated, and this consumed amount is converted to the amount of oxygen [mg-O2/L].
A reducing agent such as potassium oxalate K2C2O4 (Table 7-9) is used to quantify the remaining oxidizing agent.
7.水浄化の事例
(a) 電解鉄とリン除去
リン除去型小型浄化槽には、電解鉄法が広く用いられている。一対の鉄板を電源に接続して、電解溶出したFe2+(直ちに、酸素酸化されてFe3+に変換される。)がリン酸イオンと反応し不溶性塩Fe2PO4を生成させることにより、リンを除去する。
7. Examples of Water purification processes
(a) Iron electrodes and phosphorus removal
The iron electrodes method is widely used for small septic tank of phosphorus removal type. When a pair of iron plates are connected to a power source, electrolyzed Fe2+ is immediately oxygen-oxidized and converted to Fe3+ which reacts with phosphate ion to form the insoluble salt Fe2PO4.
(b) オゾンと上水・下水
オゾンO3は、非常に強い酸化力を有すること(表6-13)および水に溶けやすいことから、水中の物質を酸化し、脱色・脱臭・殺菌などに多用されている。トリハロメタンなども生成せず、水中で自然分解するので安全な方法といえる。
(b) Ozone and tap-water/sewage
Ozone O3 has a very strong oxidizing power (Table 6-13) and is easily soluble in water, so it oxidizes substances in water and is widely used for decolorization, deodorization and sterilization. It is a safe method as it does not generate trihalomethanes and spontaneously decomposes in water.
(c) 生物による酸化還元反応
表7に示すように、有機化合物の酸化還元反応は理論的な水の電気分解(水素発生:0.00~酸素発生:1.23V)が起こらない範囲内にある。有機物の酸化還元反応ついては、通常の条件下ではその反応が進行しないことが多い。上記COD測定でも触れたが、二クロム酸カリウムK2CrO7による有機物の酸化では、硫酸酸性の沸騰水または還流(105℃)で1時間程度、反応させると有機物がほぼ完全にCO2にまで酸化分解されるが、同じ条件下でも過マンガン酸カリウムKMnO4では有機物を完全には酸化分解できない。
しかしながら、好気性生物による酸化分解反応や嫌気性生物によるメタン発酵(複雑な細菌群による共生反応)などに見られるように、有機物の酸化還元反応が常温で進行する。植物による光合成反応では、常温で炭酸ガスを還元して有機物を合成する。また、温泉や深海熱水口では、硫化水素(表6-2)をエネルギー源とするバクテリアが存在し、深海熱水口ではバクテリアを捕食する生物やバクテリアを特殊な器官に付着/保持して有機物を摂取している生物などが生息している。これらの反応は複雑な生物化学反応から構成されており、その機構は極めて複雑であり、生命とは凄いものである。
(c) Redox reactions by living organisms
As shown in Table 7, the redox potencials of the general organic compounds are not within the range where theoretical electrolysis of water (hydrogen generation: 0.00 to oxygen generation: 1.23V), herefore, the redox ractions in their aqueous soution do not proceed under normal conditions. As mentioned in the above COD measurement, the extremly strong oxidizing organic substances such as potassium dichromate K2CrO7 are able almost to completetly oxidize and decopose the organic substances to CO2 only in boiling sulfuric acid aqueous solution with reflux (105°C) for an hour. However, even under the same conditions, potassium permanganate KMnO4 cannot completely oxidatively decompose organic substances to CO2.
However, as seen in oxidative decomposition reactions by aerobic organisms and methane fermentation by anaerobic organisms (symbiotic reactions by complex bacterial groups), redox reactions of organic substances proceed at room temperature. In the photosynthetic reaction by plants, carbon dioxide is reduced at room temperature to synthesize organic substances. In addition, there are bacteria that use hydrogen sulfide (Table 6-2) as an energy source at hot springs and deep-sea hot water outlets, and at the deep-sea hot water outlets, there are special organisms and bacteria that prey on bacteria. Inhabited by organisms that attach to/hold organs and ingest organic substances. These reactions are composed of complicated biochemical reactions, the mechanism is extremely complicated, and life is amazing.
8.電極の機能と名称
アノード・カソードの定義
電気回路の電極
電極とは、受動素子、真空管や半導体素子のような能動素子、電気分解の装置、電池などにおいて、その対象物を働かせる、あるいは電気信号を測定するなどの目的で、電気的に接続する部分をいう。
電気化学(電気分解)やダイオード(真空管、半導体素子)では、外部回路から電流(i)が流入する/外部回路に電子(e-)が流出する電極をアノード(anode)と呼んでいる。
反対に、外部回路に電流が流出する/外部回路から電子が流入する電極をカソード(cathode)と呼んでいる。
(アノード、カソードの語はファラデーにより命名され、ギリシャ語で’上り口’を意味する’anodos’と’下り口’を意味する’cathodos’に由来する。)
電気分解/電池放電の電極
電解液(固体の場合もある)中の活性物質から電子(e-)を受け取り、外部回路に電子を放出する電極がアノードで、酸化反応が起きる。外部回路から電子が流入し、電解液中の活性物質に対して電子を供与する電極がカソードで、還元反応が起きる。
なお、Pt・Cなどの不活性電極では、電解液中の活性物質と作用して電子移動(受授)のみが進行する。しかし、Fe・Pbなどの活性アノード電極では、電極が自ら電解液中の活性物質へ電子を放出(供与)し、イオンとして溶解するか、または、電解液中の物質と反応して不溶性の塩・酸化物などを形成する。
8. Function and name of electrode
Definition of anode/cathode
Electrode of electric circuit
An electrode is a passive element, an active element such as a vacuum tube or a semiconductor element, an electrolysis device, a battery, or the like, which is a portion electrically connected to the object for the purpose of working or measuring an electric signal.
In electrochemistry (electrolysis) and diodes (vacuum tubes, semiconductor devices), the electrode where the current (i) flows in from the external circuit and electrons (e-) flow out to the external circuit is called the anode.
On the contrary, the electrode through which current flows out of the external circuit and electrons flow in from the external circuit is called the cathode.
(The terms anode and cathode are named Faraday, and are derived from the Greek words’anodos’ meaning’upstream’ and’cathodos’ meaning ‘downstream’.)
Electrolysis/battery-discharge electrodes
An oxidation reaction occurs when the electrode that receives electrons (e-) from the active substance in the electrolytic solution (including solid) and releases the electrons to the external circuit is the anode. Electrons flow from an external circuit, and an electrode that donates electrons to the active substance in the electrolyte is the cathode, and a reduction reaction occurs.
Note that, with an inert electrode such as Pt or C, only the electron transfer proceeds by acting with the active substance in the electrolytic solution. However, in an active anode electrode such as Fe/Pb, the electrode itself releases (donates) electrons to an active substance in the electrolytic solution and dissolves as an ion or reacts with a substance in the electrolytic solution to form an insoluble salt or oxide.
本サイトの表記
本サイトでは「電極の機能と名称」を下記のように表記する。
アノード・カソード
アノード = 外部回路に電子を放出する電極 = 電解液中の活性物質から電子を受容する電極 = 酸化反応が起こる電極
カソードは上記事項に対して、それぞれ逆に対応する。
正極と負極
電位により極性を定義する場合は、電位が高い方を正極(+)(positive electrode)、低い方を負極(-)(negative electrode)と呼ぶ。両極に抵抗等の負荷を掛けて接続したとき、外部電流は正極(+)から負極(-)へ、電子は負極(-)から正極(+)へ流れる。
電池と電解
正極/負極とアノード/カソードは、電池と電気分解(電解)では対応が逆になる。
電解(充電): 正極 = アノード、負極 = カソード
電池(放電): 正極 = カソード、負極 = アノード
これは、電解(充電)の時には正極へ電流が流れ込み、電池(放電)では正極から電流が流れ出すことに対応する。例えば、二次電池である鉛蓄電池のPbO2極は、充電時も放電時も正極であるが、充電時は電流が流れ込むアノードとなり酸化され、放電時は電流が流れ出すカソードとなり還元される。
陽極と陰極
陽極・陰極の用語は、電流の方向(酸化・還元の方向)による(アノード・カソードの直訳)とする流儀と、電位の高低による流儀があり、混乱している。これに対して、正極・負極の用語は、電位の「高い・低い」の区別として定着している。高校化学では、電池の場合「正極・負極」、電気分解の場合「陽極・陰極」と呼んでいる。
Notation on this site
On this site, “electrode functions and names” are described as follows.
Anode/cathode
Anode = Electrode that emits electrons to an external circuit = Electrode that accepts electrons from the active substance in the electrolyte = Electrode where oxidation reaction occurs
Cathode responds to the above items in reverse.
Positive and negative electrodes
When the polarity is defined by the potential, the higher potential is called the positive electrode (+) and the lower potential is called the negative electrode (-). When a load such as a resistance is applied to both electrodes and connected, an external current flows from the positive electrode (+) to the negative electrode (-), and electrons flow from the negative electrode (-) to the positive electrode (+).
Battery and electrolysis
The correspondence between positive/negative electrodes and anode/cathode is reversed in batteries and electrolysis (electrolysis).
Electrolysis (charging): Positive electrode = Anode, Negative electrode = Cathode
Battery (discharge): Positive electrode = cathode, negative electrode = anode
This corresponds to the current flowing into the positive electrode during electrolysis (charging) and the current flowing out from the positive electrode during battery (discharging). For example, the PbO2 electrode of a lead acid battery, which is a secondary battery, is a positive electrode during charging and discharging, but becomes an anode through which current flows in during charging and is oxidized, and becomes a cathode through which current flows out during discharging and is reduced.
Anode and cathode
The terminology for anodes and cathodes is confusing in Japan, because there have been two styles: (1) depending on the direction of current (direction of oxidation/reduction) (direct translation of anode/cathode) and (2) depending on the high or low of the potential.
On the other hand, the terms positive electrode and negative electrode are well established as the distinction between “high and low” in potential. In Japanese high school chemistry, “positive and negative” electrodes are used for batteries and “anode and negative” electrodes for electrolysis.
掲載日:2018年01月24日
更新日:2020年01月08日(目次を追記、「8.電極の機能と名称」を追記)
更新日:2020年06月20日(英語版を掲載)
Published: 2018/01/24
Updated: January 08, 2020 (Added table of contents and “8. Functions and names of electrodes”)
Updated: June 20, 2020 (English version posted)